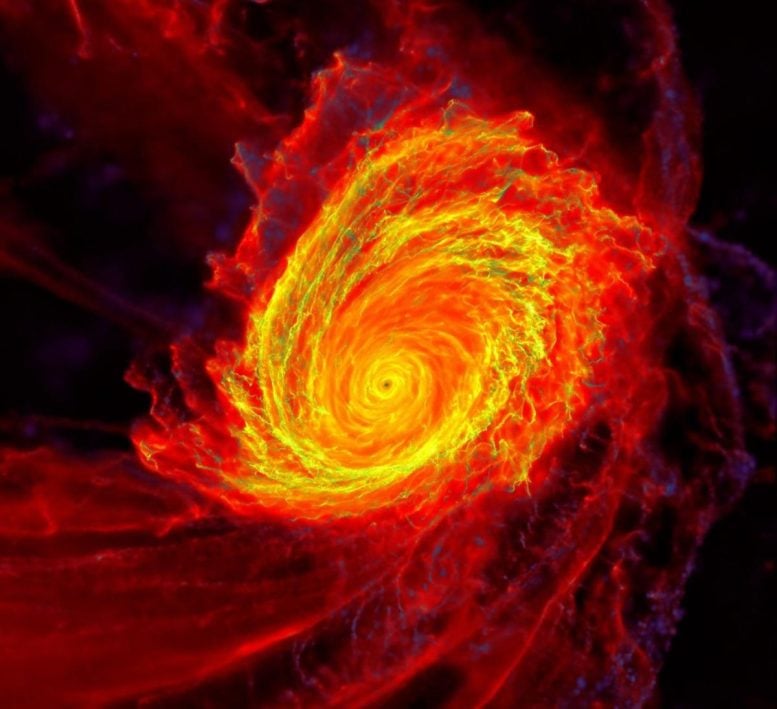
Zdumiewająca symulacja pokazuje, że pola magnetyczne tworzą puszyste, a nie płaskie dyski akrecyjne wokół supermasywnych czarnych dziur, zmieniając nasze rozumienie[{” attribute=”” tabindex=”0″ role=”link”>black hole dynamics.
A team of astrophysicists from Caltech has achieved a groundbreaking milestone by simulating the journey of primordial gas from the early universe to its incorporation into a disk of material feeding a supermassive black hole. This innovative simulation challenges theories about these disks that have persisted since the 1970s and opens new doors for understanding the growth and evolution of black holes and galaxies.
“Our new simulation marks the culmination of several years of work from two large collaborations started here at Caltech,” says Phil Hopkins, the Ira S. Bowen Professor of Theoretical Astrophysics.
Bridging the Scale Gap in Cosmic Simulations
The first project, called FIRE (Feedback in Realistic Environments), investigates large-scale cosmic phenomena such as galaxy formation and collisions. The second, known as STARFORGE, focuses on smaller-scale processes, like star formation within individual gas clouds. “But there was this big gap between the two,” Hopkins explains. “Now, for the first time, we have bridged that gap.” Achieving this required a simulation with over 1,000 times the resolution of any previous efforts in the field.
To the team’s surprise, as reported in The Open Journal of Astrophysics, the simulation revealed that magnetic fields play a much larger role than previously believed in forming and shaping the huge disks of material that swirl around and feed the supermassive black holes. “Our theories told us the disks should be flat like crepes,” Hopkins says. “But we knew this wasn’t right because astronomical observations reveal that the disks are actually fluffy—more like an angel cake. Our simulation helped us understand that magnetic fields are propping up the disk material, making it fluffier.”
Superkomputerowe dyski akrecyjne czarnych dziur
W nowej symulacji naukowcy przeprowadzili tak zwane „super przybliżenie” pojedynczej supermasywnej czarnej dziury, potwornego obiektu leżącego w sercach wielu galaktyk, w tym naszej własnej[{” attribute=”” tabindex=”0″ role=”link”>Milky Way. These ravenous, mysterious bodies contain anywhere from thousands to billions of times the mass of the Sun, and thus exert a huge effect on anything that comes near.
Astronomers have known for decades that as gas and dust are pulled in by the tremendous gravity of these black holes, they are not immediately sucked in. Instead, the material first forms a rapidly swirling disk called an accretion disk. And as the material is just about to fall in, it radiates a huge amount of energy, shining with a brilliance unmatched by just about anything in the universe. But much is still not known about these active supermassive black holes, called quasars, and how the disks that feed them form and behave.
While disks around supermassive black holes have been imaged previously—the Event Horizon Telescope imaged disks circling black holes at the heart of our own galaxy in 2022 and Messier 87 in 2019—these disks are much closer and more tame than the ones that churn around quasars. To visualize what happens around these more active and distant black holes, astrophysicists turn to supercomputer simulations. They feed information about the physics at work in these galactic settings—everything from the basic equations that govern gravity to how to treat dark matter and stars—into thousands of computing processors that work in parallel. This input includes many algorithms, or series of instructions, for the computers to follow to recreate complicated phenomena. So, for example, the computers know that once gas becomes dense enough, a star forms. But the process is not that straightforward.
“If you just say gravity pulls everything down and then eventually the gas forms a star and stars just build up, you’ll get everything wildly wrong,” Hopkins explains. After all, stars do many things that affect their surroundings. They shine radiation that can heat up or push surrounding gas. They blow winds like the solar wind created by our own Sun, which can sweep up material. They explode as supernovae, sometimes launching material clear out of galaxies or changing the chemistry of their surroundings. So, the computers must know all the ins and outs of this “stellar feedback” as well, as it regulates how many stars a galaxy can actually form.
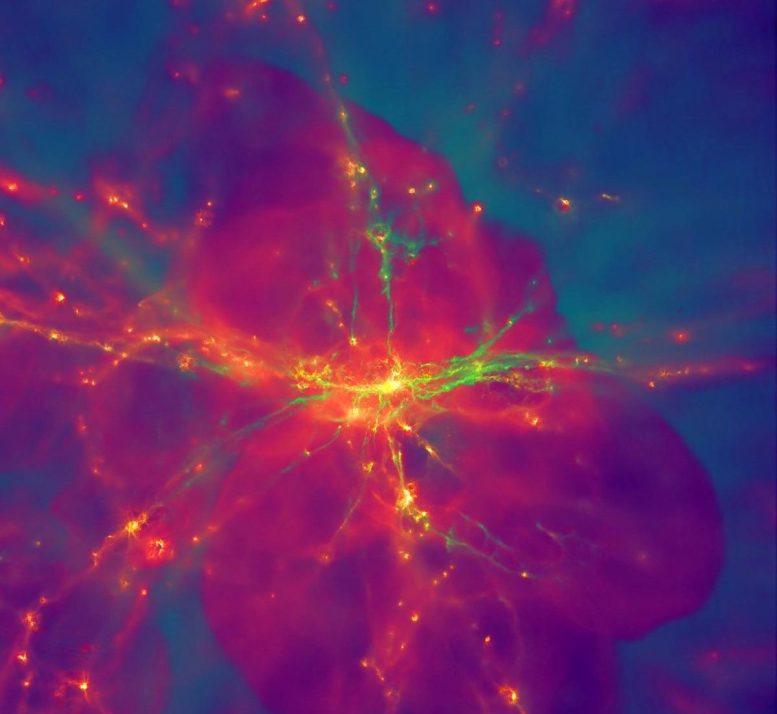
Unveiling New Insights on Black Hole Dynamics
But at these larger scales, the set of physics that are most important to include and what approximations can be made differ from those at smaller scales. For example, on the galactic scale, the complicated details of how atoms and molecules behave are extremely important and must be built into any simulation. However, scientists agree that when simulations focus on the more immediate area around a black hole, molecular chemistry can be mostly ignored because the gas there is too hot for atoms and molecules to exist. Instead, what is exists there is hot ionized plasma.
Creating a simulation that could cover all the relevant scales down to the level of a single accretion disk around a supermassive black hole was a huge computational challenge—one that also required a code that could handle all the physics. “There were some codes that had the physics that you needed to do the small-scale part of the problem and some codes that had the physics that you needed to do the larger, cosmological part of the problem, but nothing that had both,” Hopkins says.
The Caltech-led team used a code they call GIZMO for both the large- and small-scale simulation projects. Importantly, they built the FIRE project so that all the physics they added to it could work with the STARFORGE project, and vice versa. “We built it in a very modular way, so that you could flip on and off any of the pieces of physics that you wanted for a given problem, but they were all cross-compatible,” Hopkins says.
This allowed the scientists in the latest work to simulate a black hole that is about 10 million times the mass of our Sun, beginning in the early universe. The simulation then zooms in on that black hole at a moment when a giant stream of material is torn off a cloud of star-forming gas and begins to swirl around the supermassive black hole. The simulation can continue zooming in, resolving a finer area at each step as it follows the gas on its way toward the hole.
Nowa symulacja wlatuje w plątaninę łączących się galaktyk, ostatecznie zbliżając się do aktywnej supermasywnej czarnej dziury lub kwazarotoczony wirującym dyskiem materiału zwanym dyskiem akrecyjnym. Włóknisty strumień gazu został nawinięty na dysk, wtłaczając go z szybkością wystarczającą do zasilania najjaśniejszych znanych kwazarów we wszechświecie. Pod koniec symulacji pola magnetyczne wyrywają moment pędu z wirującego dysku, co pozwala materii wirować coraz dalej, aż dotrze do horyzontu zdarzeń czarnej dziury, skąd nie może uciec. Podczas tej symulacji, która przedstawia jeden moment w czasie, skala powiększa się miliard razy. Kolory pokazują gęstość gazu, przy czym jaśniejsze kolory reprezentują wyższe gęstości. Źródło: grupa Caltech/Phil Hopkins
Rewizja teorii wpływu magnetycznego na dyski akrecyjne
„W naszej symulacji widzimy, jak dysk akrecyjny tworzy się wokół czarnej dziury” – mówi Hopkins. „Bylibyśmy bardzo podekscytowani, gdybyśmy właśnie zobaczyli ten dysk akrecyjny, ale bardzo zaskakujące było to, że symulowany dysk nie wygląda tak, jak myśleliśmy od dziesięcioleci, że powinien wyglądać”.
W dwóch przełomowych artykułach z lat 70. opisujących dyski akrecyjne zasilające supermasywne czarne dziury naukowcy przyjęli, że ciśnienie termiczne – zmiana ciśnienia spowodowana zmianą temperatury gazu w dyskach – odgrywa dominującą rolę w zapobieganiu zapadaniu się takich dysków pod wpływem ogromnej grawitacji, jakiej doświadczają w pobliżu czarnej dziury. Przyznali, że pola magnetyczne mogą odgrywać niewielką rolę we wzmacnianiu dysków. Z kolei nowa symulacja wykazała, że ciśnienie pól magnetycznych takich dysków było w rzeczywistości 10 000 razy większe niż ciśnienie wytwarzane przez ciepło gazu.
„Zatem dyski są prawie całkowicie kontrolowane przez pola magnetyczne” – mówi Hopkins. „Pola magnetyczne spełniają wiele funkcji, a jedną z nich jest podpieranie dysków i powodowanie puchnięcia materiału”.
Odkrycie to zmienia szereg przewidywań, jakie naukowcy mogą poczynić na temat dysków akrecyjnych, takich jak ich masa, gęstość i grubość, szybkość przemieszczania się materiału z nich do czarnej dziury, a nawet ich geometria (np. czy dyski mogą być przekrzywione).
Patrząc w przyszłość, Hopkins ma nadzieję, że ta nowa możliwość wypełnienia luki w skalach symulacji kosmologicznych otworzy wiele nowych kierunków badań. Na przykład, co dzieje się szczegółowo, gdy dwie galaktyki łączą się? Jakie typy gwiazd powstają w gęstych obszarach galaktyk, gdzie warunki różnią się od tych w sąsiedztwie naszego Słońca? Jak mogła wyglądać pierwsza generacja gwiazd we wszechświecie? „Jest mnóstwo do zrobienia” – mówi.
Nowa symulacja została szczegółowo opisana w artykule zatytułowanym „FORGE’d in FIRE: Resoling the End of Star Formation and Structure of AGN Acretion Disks from Cosmological początkowe warunki”, który ukazuje się w czasopiśmie Otwarty dziennik astrofizyki.
Odniesienie: „FORGE’d in FIRE: Resoling the End of Star Formation and Structure of AGN Accretion Disks from Cosmological początkowe warunki” autorstwa Philipa F. Hopkinsa, Michaela Y. Grudica, Kung-Yi Su, Sarah Wellons, Daniela Angles-Alcazara, Ulrich P. Steinwandel, David Guszejnov, Norman Murray, Claude-Andre Faucher-Giguere, Eliot Quataert i Dusan Keres, 14 marca 2024 r., Otwarty dziennik astrofizyki.
DOI: 10.21105/astro.2309.13115
Dodatkowi autorzy artykułu to Michael Grudic (doktorat ’19) z Carnegie Observatories, Kung-Yi Su (doktorat ’19) z Harvard University, Sarah Wellons z Wesleyan University, Daniel Angles-Alcazar z University of Connecticut i Flatiron Institute, Ulrich Steinwandel z Flatiron Institute, David Guszeinov (doktorat ’18) z University of Texas w Austin, Norman Murray (BS ’79) z University of Toronto, Claude-Andre Faucher-Giguere z Uniwersytet Północno-ZachodniEliot Quatert z Uniwersytet Princetoni Dusan Keres z Uniwersytetu Kalifornijskiego w San Diego. Praca Hopkinsa była wspierana dzięki funduszom z National Science Foundation i NASA.